This is the best entry point for this slimmed-down second series of three posts. The other two are supporting material (Part 1 covers the contour-canal reservoir scheme, and Part 2 looks at hydrodynamics).
Summary
The goal: provide large-scale, long-duration storage sufficient to reach 100% grid carbon elimination throughout the Western states, as quickly as humanly possible, at a competitive cost, using established technologies. Pumped hydroelectric storage “remains the largest contributor to U.S. energy storage with an installed capacity of 21.6 GW, or roughly 95% of all commercial storage capacity in the United States1,” so it’s certainly established; can we build more?
The obstacles: Some energy experts sound almost wistful when they talk about pumped storage. Despite its proud history and many virtues, it’s often viewed as a legacy technology: it takes far too long to build; all the good sites are taken; and most of all, new sites can’t be approved due to environmental and other objections.
The new paradigm: Don’t focus on sites that would be good for pumped hydro as currently conceived. Instead, start by looking for places that are no good for anything else. The unavoidable requirements for surface pumped storage are large elevation differences, and large land areas (due to the modest energy density of all gravity-based systems). Then on to the real criterion: a lack of competing land uses of any kind—residential, commercial, wildlife habitat, recreation, history, religious significance—anything. In other words, find the least-loved places on earth (knowing that every place is loved by someone). Then try to adapt pumped hydro to work in those places, because they’re the ones you might be able to get.
If such places can’t be found, or if pumped storage just won’t work in them, then it’s time to face the fact (as many already have) that there will not be a new Golden Age for pumped storage; it will continue as a significant, but niche technology, making a small contribution to reducing our emissions.
I’ve spent my adult life in the American West, and have always been drawn to its emptiest and least-loved places, so that’s where I started looking.
The solution: Closed-loop pumped hydroelectric storage, far from any existing watercourses—conventional in most respects, but with reservoirs consisting of polymer-lined earthen canals with floating covers, situated along contours (lines of constant elevation). I call this Encapsulated Pumped Storage (EPS).
Why canals on contours? (1) the need for flat land or dammable canyon topography is eliminated; (2) construction is fast, using common excavation tools and techniques; (3) embodied carbon is extremely low; (4) the system can start small and be expanded incrementally; (5) design is standardized, for economy of scale and cost improvements over time.
Why covered canals? (1) the cover, by preventing evaporation, allows operation in hot, dry deserts, while reusing a one-time water allocation indefinitely; (2) that, in turn, opens up sites that have no competing economic uses, and which are on the more easily developed end of the spectrum of ecological, cultural, and other non-monetary values.
Water storage aside, the system is conventional, which means there are well-established vendors for turbines, generators, valves, and all other components, and widespread experience in putting systems together.
The Ragtown Site
In this post I’ll explain my proposal by describing a real site in the Mojave Desert—consisting of one large alluvial slope covering a significant range of elevation—and how this site could be exploited for Encapsulated Pumped Storage.
I’m abandoning my previous reticence about real sites in this case, for two reasons:
1. This isn’t a particularly great site for EPS, because the head (maxing out at about 360 meters) is lower than optimal, and the shallow slope means the penstocks will need to be relatively long. There are many other sites in the Mojave that are better in these respects. I believe there is no shortage of good sites for EPS, and so rather than focusing on the very best candidate I can find, I think it makes more sense to look at a mid-range one.
2. This site is right in the middle of Twentynine Palms Marine Corps Base, so building EPS on it is probably out of the question to begin with. (Or maybe I’m too pessimistic: the U. S. military services, which consume vast amounts of energy, have ambitious renewable-energy goals2.)
I’m going to call this site “Ragtown” after a ghost town nearby, a remnant of gold and copper mining in the late 1800s. Here’s the general location (orange dot):
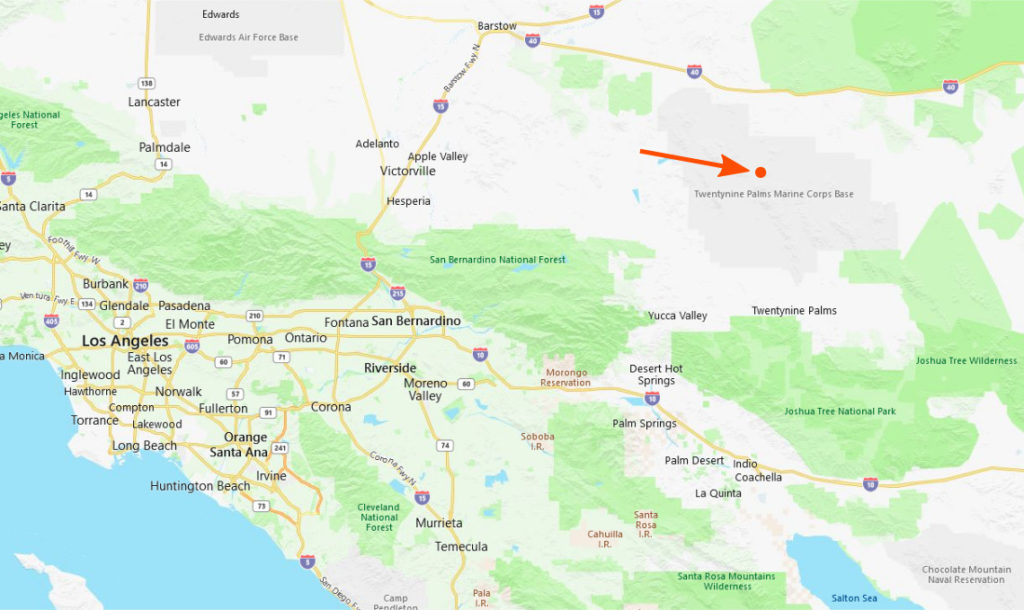
Switching to Google Earth, here is the area of interest. This view is toward the southwest, with the Pacific Ocean visible on the horizon:
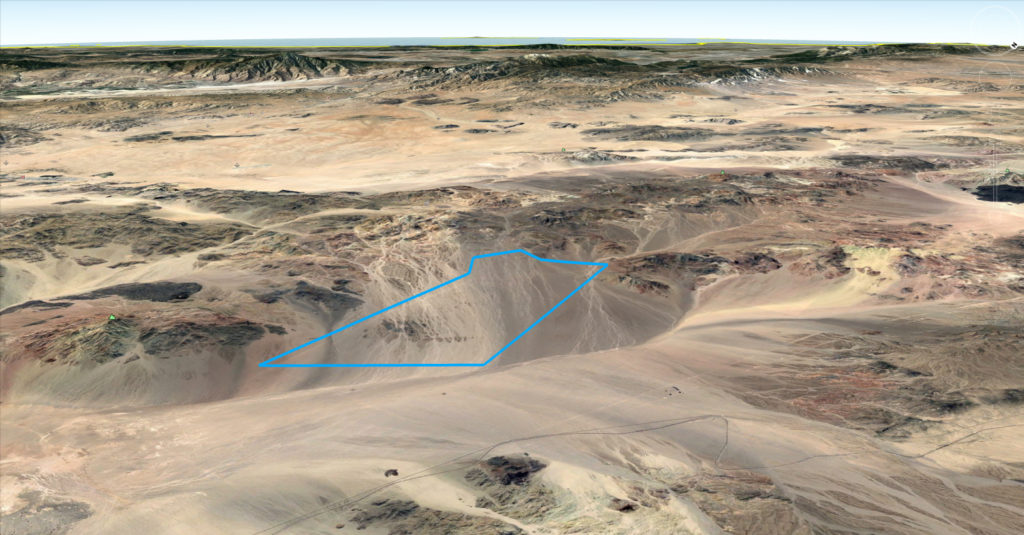
This side view that shows the slope is as flat and featureless as a slightly tilted table:
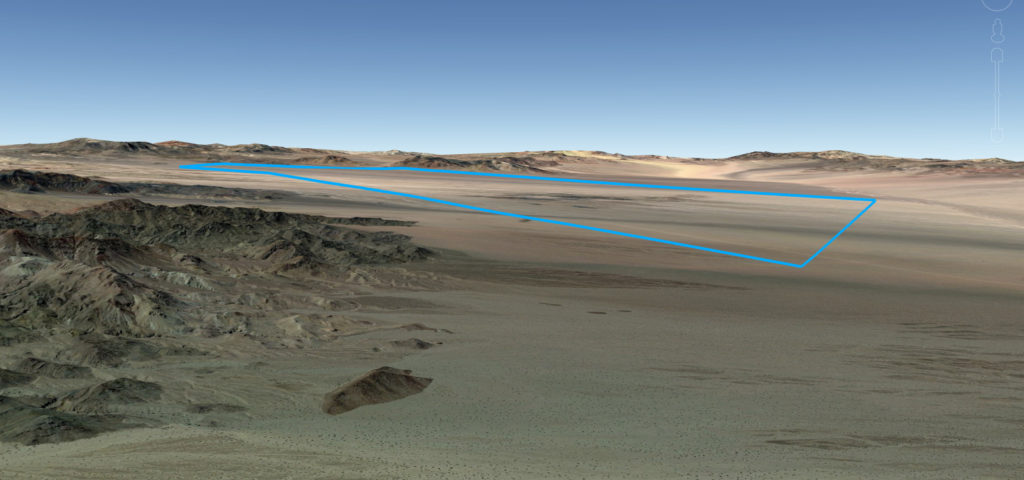
One reason to show these images is to try to convey how astonishingly empty this place is. In the late 1800s, many parts of the Mojave Desert had something that was of interest to the commercial world: gold, copper, and other minerals. These were extracted within a few decades, leaving behind some place names and little else. There are roads and rail lines passing through, and probably some damage from military exercises. But this particular spot lacks even those features. No one has successfully farmed or settled in a place like this. It’s hard to imagine that Native Americans, passing through to follow game or gather plants, would stay for long (or would visit in the summer). And this utter emptiness and lack of utility is an increasingly scarce resource.
Zooming in from above, it looks like this:
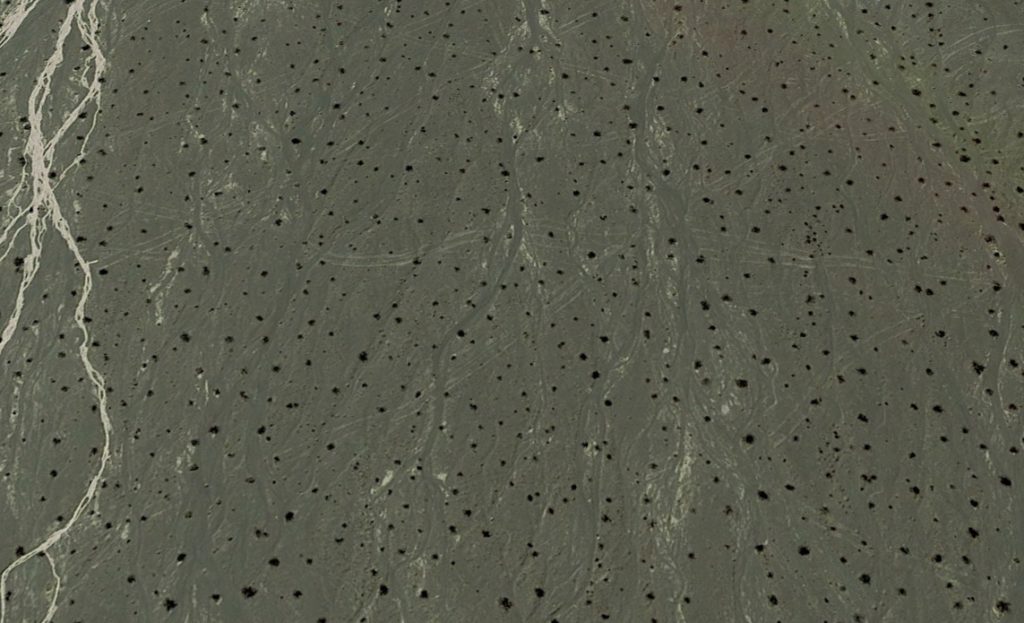
There are occasional braided washes caused by sporadic rains, and shrubs that, because of their regular spacing, make me think of creosote bushes3. It looks like four-wheelers enjoy using this open space, making tracks of indeterminate age.
Site Characteristics
The area of interest is about 30 square miles (7,800 hectares) in area, a little under 10 miles (16 km) across from east to west, and consists of one large, gently sloped alluvial fan. The area marked is the same as in the images above. Notice that the contour lines are straight and regular:
The slope is very gradual, about 3%, which (as noted) leads to a long penstock run, but will make construction of the canals (described here) very easy. Shallow trenching, installation, and covering of the penstock and tailrace should also be very straightforward.
The marked area is an undisturbed alluvial fan, with no arroyos that would need filling in. (The Mojave Desert is the driest region in the United States; the nearby town of Twentynine Palms gets an average of 4.5 inches of rainfall annually, compared to a national average of about 38 inches.)
Ecological, religious, and cultural concerns
There’s no point designing a system that shouldn’t be built, or won’t be built, because it doesn’t respect its neighborhood, the people who will live near it, the people who were there before it, or the plants and animals who were there before the people.
The motivation to build a grid storage system is to reduce fossil fuel use, and make a dent in the global heating crisis that is already causing wholesale destruction of ecosystems and cultures around the world. Still, “destroying the planet in order to save it” is not a viable strategy. Our technological interventions to slow global heating almost always have local impacts that can’t just be waved away (though it’s been tried). Grid-scale solar arrays are a good example: we need them, but they drastically change the ecosystems that they cover. It’s a balancing act, and it isn’t just about the sheer quantity of life affected, though that matters too. Endangered species and ecosystems are given extra weight. For that reason, much of the Mojave Desert is under one form of ecological protection or another. Some of these areas are likely forever off-limits for any sort of development, while others may be negotiable. Complete transparency, and educating stakeholders and the public about the benefits of an EPS system, will always be crucial for winning approval.
Pumped hydro runs on water, and in today’s world there is no possible use of water that isn’t environmentally significant. Interfering with natural waterways and their aquatic life is, to many, unthinkable; there’s more public support for removing existing dams than for building new ones. Closed-loop pumped storage, particularly with reservoirs built from scratch on formerly dry land, is a big step forward. But in dry climates, it still needs an annual water allotment to make up for evaporation and seepage; and if it sits in a drainage area, whatever water falls into that area each year is water that may not be going where it used to go (i.e. into an aquifer).
EPS takes the last step and fully encloses the water it uses (hence the name), isolating it from the environment. With careful management, water loss per year can be very close to nil. And the narrow, canal-shaped reservoir design makes it easier for runoff from rainfall to be routed around the reservoirs. (What rain does fall onto the canals will be removed by pumps and distributed back onto the land.)
The ability of EPS to run on low-quality or even contaminated water is discussed below.
Moving on from water to other issues, I think it’s useful to compare an EPS with one of the large solar arrays that are popping up in many parts of the Mojave. The water-storage canals, like solar arrays, are substantial in area, which would be acreage essentially snipped out of the environment and made unavailable to plant and animal life. One difference is that the canal surface is meant to reflect away as much heat as possible, but the solar array has a more complex effect4 5. Canals aside, impacts will be minor if the penstocks are covered by a low mound of soil and rocks, as proposed in the first part of this series.
Beyond ecosystem values, many places are sacred or culturally significant to the people who’ve lived there the longest, Native Americans. Discussions with the tribes themselves will be the best way to avoid affecting those places—given the siting flexibility of EPS, there may be another place to build. Of course, when considering a site that is actually on tribal land, tribal government must be in the loop from the earliest planning stages.
Finally, many believe that empty places should be left untouched simply because so few of them remain. This is a reasonable view, but only if applied uniformly: it should not be OK to allow vast areas to be consumed for fossil fuel and mineral extraction, while saying no to more modest land uses that are meant to slow down the greater destruction.
If, after consideration of all the above factors, permission to use this particular site is granted, what can we build there?
Phase One
A 3-kilometer-long canal, 250 m wide bank to bank, can be built at 632 m elevation for upper storage. (It may look something like this, only white.) Here we are looking directly down at the upper end of the site—the top of the picture is the upslope direction (west). The green rectangle is the canal location, and the purple line is the location for the penstock, which will pump water into and out of the canal near its center6. The thin black line is a contour at 632 m, showing that excavating this canal will be almost as easy as if it were in Kansas. The usable water capacity of this canal is 18.75 million cubic meters (which is 5 billion gallons, or 15,000 acre-feet).
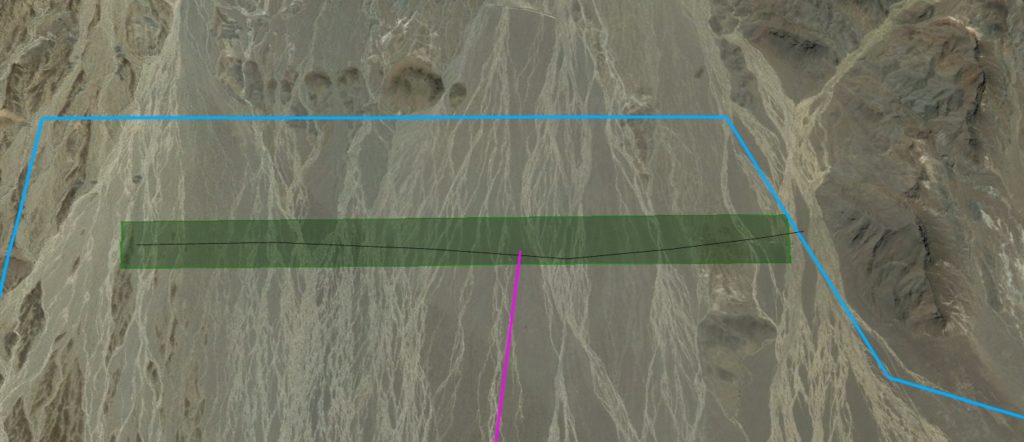
A similar canal can be built at 270 m for lower storage. The head would be 632 – 270 = 362 m. (As the black 270 m contour shows, there’s room there for at least 6 km of canal, but we would only build 3 km to match the upper canal.)
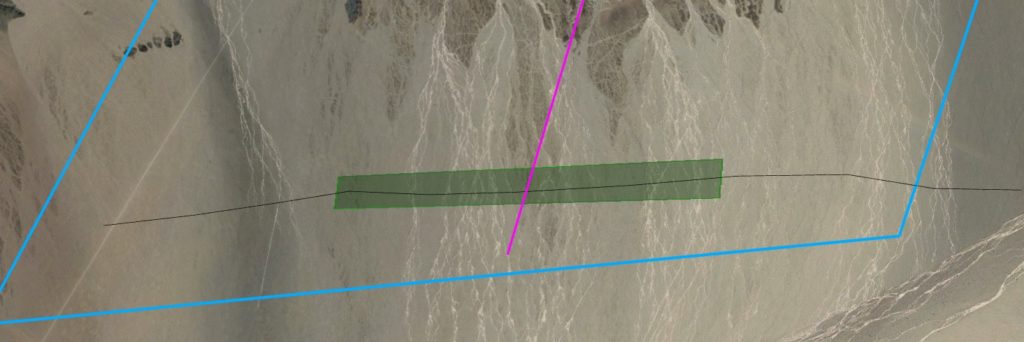
A penstock/tailrace combination about 11 km in length joins the two canals. Because the whole site is sloped, there’s no headrace at all—the upper canal is joined directly to the penstock. The powerhouse will be located very close to the lower canal, connected by a minimal tailrace. From the previous post, where I analyzed hydrodynamic losses in penstocks, this is the “low head” scenario, and the chart there shows that we would need a penstock of about 10 meters (33 feet) in diameter to achieve 90% round-trip penstock efficiency at 1 GW peak power, and even bigger if the power requirement is greater. This large penstock diameter—the price paid for a gradual slope with low head—is the main reason I described the site’s topography as less than ideal. This site might be a good place for the strategy I mentioned in the previous post: plan for two penstocks (both large, but less than 10 meters), but only build one at first.
Here is the full overhead view. This is looking directly down; the penstock seems skewed because it is—the canals have to follow the contours exactly, but to get the lower canal at a slightly lower elevation, the two canals are offset in the cross-slope direction.
Here is the cross section (not to scale):
Phase 1 energy and power
I calculated here that the larger, 250-meter-wide canal design stores 13.1 GWh of energy per kilometer of canal, at 1 km head. So, at 0.362 km head and a length of 3 km, the theoretical energy storage from this pair of canals would be:
This is 77 Hornsdales, or 57% more than the famous Dinorwig Power Station in the U.K., which stores 9.1 GWh7. In other words, I believe it would be, by far, the largest energy storage deployment in the Western Hemisphere since the 1980s. The location is very convenient, only about 65 miles from the edge of the power-hungry Los Angeles metro area.
As for the peak generating and storing power capacity, a study would have to be done to optimize this to the regional market. Ten hours’ duration is a popular rule of thumb for new energy storage proposals, so as a starting point, we could divide 14.2 by 10 to get 1.42 gigawatts of peak power. (For comparison, Dinorwig has 1.8 gigawatts.) The point is to pay for the amount of power that will be useful, but not more. See this post for how I used simulation to address this question.
Phase Two
Once we’ve gotten the initial 14.2 GWh system online, the site has lots of room for expansion, all on the same smooth, construction-friendly 3% slope. At the upper end, the alluvial fan narrows above 630 meters elevation, so we would probably prefer to move downhill, where the fan widens and we can build longer canals. At the least, we could build two more 3-km-wide canals below the first, at perhaps 612 and 590 m elevations. (In fact, these can be longer than 2 km—more like 3.5 and 3.75 km in length, respectively.)
At the bottom, things are even more accommodating. We could just extend our initial 3 km canal (at 270 km) to 7 km, or we could build two new canals downslope. The advantage of building new canals is that we could then have three pairs of canals, each having about the same head. Here’s an overhead view of the expanded system:
So, very conservatively, we could add two more subsystems of the same capacity as the first, each with its own penstock(s) and powerhouse (but using a shared control and grid management system, maintenance, security, and other functions). This would increase both energy and power by a factor of at least 3, for a total of 42.6 GWh and 4.26 gigawatts, making the Ragtown facility the largest energy storage system in the world, both by energy (exceeding Raccoon Mountain’s 36 GWh) and by power (exceeding Bath County’s 3 GW).
(The Fengning Pumped Storage Power Station in China, now under construction, will have a maximum power of 3.6 GW. I haven’t found published energy numbers for this system, but the information at Wikipedia claims 40 million cubic meters of usable water volume and 424 meters head, which at 75% round-trip efficiency would work out to 36 GWh.)
Water supply
Phase 1 would require one canal’s worth of water, 18.75 million cubic meters. (This is 4.95 billion gallons, or 15,200 acre-feet.)
Though the water in EPS should last indefinitely, getting that initial fill is a challenge that will be unique to each site. It definitely helps that EPS water doesn’t need to be potable, or even treatable to be safe for human use. The water can contain a variety of non-particulate pollutants, from solvents to heavy metals, as long as they are compatible with the plastic and steel that contacts the water. (Water that’s very brackish—salty—corrodes steel, so such water could only be used if diluted with fresher water.) In general, there are many more water wells that produce non-potable water than produce potable water, and non-potable water is not as valuable. A well field at the site, or close enough for a temporary pipeline to the lower storage, is the easiest solution.
If that isn’t possible, and we need to bring in water, the Ragtown site fortunately has a railway line less than 5 miles from the lower canal(s)8. A temporary pipeline would close the gap. Trucking in water would probably be prohibitively expensive.
If we can use produced water from fracking operations, it might be cheaper for fracking operators to bring the water to us at their expense than to pay for the level of treatment the water would otherwise require before disposal. Otherwise, the biggest challenge will be to convince the public that this amount of groundwater, amortized over 50 years or more of benefit, is worthwhile. The fact that makeup water won’t be needed each year due to evaporation should help make the case.
Cost
This design is optimized for fast construction and low embodied carbon footprint. The decisions that result from these criteria (minimal modifications to the site, and as little manufactured material as possible) should also favor low cost, but more detailed studies and experience would be needed to accurately estimate all three numbers (time, money, and carbon).
In an earlier post I looked at historical storage costs and concluded that $75 per kilowatt-hour would be an “excellent” price, comfortably lower than current full-system costs for lithium-ion grid storage. At that price, for Phase 1, with its 14.2 GWh of storage, the price tag would be $1.07 billion. For Phase 2, with 42.6 GWh, the bill would be $3.2 billion.
I think we might be able to do even better. The Fengning station mentioned above, per the same Wikipedia article, has a price tag of US$1.87 billion, which works out to $52 per kilowatt-hour. These numbers are unconfirmed, of course, but not unprecedented in the pumped-hydro domain.
Financial benefit
This is something I looked at in the previous series. I took a simple approach to estimating the value of an energy storage plant:
- Assume the plant is kept busy enough to turn over its rated energy capacity daily (i.e., it’s not big enough yet, relative to its market, to have capacity left over for long-duration storage).
- Assume that the energy it provides to the grid displaces natural gas, and include the cost of that much gas.
- Add in an imaginary carbon tax of $100/ton. (I don’t think carbon taxes will work—David Roberts explains the problems at his new site—but it gives me a way to put a dollar value on carbon emissions.)
In that earlier study, I worked out a cost for buying gas of 6.5 cents per kWh, and a simulated carbon tax of 4 cents per kWh. That puts the value of the Phase 1 plant at:
Based on these numbers, the Phase 1 plant would be paid off in 2 years, and yield $544 million of net value each year thereafter.
Conclusion
I started out by saying that the Ragtown site wasn’t very promising. But we can certainly build an impressive amount of energy storage here, and the uniformity of the slope would make construction easy, and expansion straightforward. The low head and long penstock length do mean that the cost per kWh will be somewhat higher here than at a number of other sites in the region. Still, if this site, and only this site, got the green light for construction tomorrow, I would say we should get to work immediately.
Previous: Encapsulated Pumped Storage, Series 2, Part 2: Hydrodynamics
- https://www.energy.gov/eere/water/hydropower-program
- A report entitled “Department of Defense Energy Management: Background and Issues for Congress” states, “DOD in accordance with 10 U.S.C. §2911(g) is required to ‘produce or procure’ 25% renewable energy (electrical and non-electrical) by FY2025.”
- My uninformed guess might even be right. This fascinating resource says “The predominant natural plant communities are Creosote bush series and Creosote bush – white bursage series.”
- See for example: The Photovoltaic Heat Island Effect: Larger solar power plants increase local temperatures. And work is being done to elevate solar panels in order to actually improve land productivity by growing crops under them, although where endangered species are concerned, it’s most likely that any disturbance is harmful.
- I should also point out that if the canal width is not too great, suspending solar panels over the canal is an interesting option. They should be above the floating cover, and should allow air circulation in between, to cool both the panels and the canal. See this study, but the economics here are not as favorable, because the solar panels don’t save any water with EPS.
- If you need an introduction to how pumped storage works, this might help.
- To my dismay, I see the Wikipedia article on Dinorwig now has a banner reading “This article may be too technical for most readers to understand.” That doesn’t bode well for what I’m trying to do here!
- There’s a railway stop named Siberia there, which was formerly a water stop and rail siding for the Santa Fe Railroad, but has been abandoned.