I’ve talked about, and perhaps at least put some bounds on, the dollar cost of EPS. Now it’s time to try to quantify the benefits. I’ll approach that from two different angles:
1. How much storage do we need, in order to stop catastrophic global heating caused by burning fossil fuels to generate electricity? (The grid is far from the only source of planet-heating emissions, of course—it doesn’t include fuel we burn in vehicles, for example. But it’s a significant subset.) This angle isn’t about money—the benefit is leaving a viable planet to future generations. That’s important enough that reasonable people will agree that we have to do it, even if it’s expensive. Call this the “because we have to” angle.
2. What is the cost/benefit ratio of EPS? This angle comes from the realistic perspective that many people will have to be persuaded that money should be spent on fighting climate change, and the less expensive it is, the easier that persuading will be. It will also help us answer practical questions that the “because we have to” approach can’t, such as: How much of our storage budget should be spent on technology A versus B versus C? And, for some particular service area, like Los Angeles or Salt Lake City, how much should we spend on solar panels versus wind turbines versus storage in order to get the best coverage of demand at the lowest cost? Call this “bang for the buck.” We can measure this either as a ratio of two dollar amounts—which means we have to assign dollars to the emissions benefits—or simply as tons of greenhouse gas emissions prevented per dollar spent.
These two angles match up pleasingly with the two main approaches that have been proposed to break the current agonizing stasis of “business as usual” and start to make real progress. The first angle, “because we have to,” corresponds to command-economy approaches like the Green New Deal, and the RPS (Renewable Portfolio Standard) laws that have been passed in a number of states, in which society, through its political system, sets explicit carbon-emission targets. (Obviously, the money to meet those targets has to come from somewhere; often it will come from tax revenues, just as we all chip in to pay for public goods like firefighting and roads.) The model here is that we know what needs to happen, and it needs to happen quicker than the free market will do it, so let’s get on with it: a wartime footing, you could say.
The second angle, “bang for the buck,” lines up pretty well with the notion of a carbon tax. This says, society (again through its political system) agrees that adding carbon to the atmosphere is bad, and sets a dollar figure on that badness; anyone who wants to emit carbon has to pay for the privilege, and that money is redistributed back into the economy in one way or another. The model here is that the free market is cleverer than the government at finding innovative ways to solve problems, so let’s just make it worth their while, and stand back.
My goal is to examine both of these angles—first from the macro perspective, by seeing what policy institutions and others who’ve studied the problem have to say. I’ll also take the micro view, examining hour-by-hour energy supply and demand for the PNM service region and the Capitan example site, and simulating the effects of adding various amounts of solar, wind, and storage.
How Much Grid Electrical Storage Do We Need?
This question is more often hand-waved than addressed head-on, so this 2017 paper’s title is refreshing: How much energy storage is needed to incorporate very large intermittent renewables? From the abstract (emphasis mine):
“It was found that when we increase energy from VRE [VRE means “variable renewable energy,” i.e. wind and solar], the use of storage and its capacity increases until we reach some threshold. After that threshold, the storage use starts to decline even if we increase the size. An optimally utilized storage of about daily average demand would be sufficient to reach grid penetration of about 90% of the total demands from VRE.”
There are a couple of important points here. First, they’re going for 90% of electricity from renewables. 100% carbon-free energy is not a realistic storage goal in the near term, because of the diminishing returns problem I discussed earlier; building enough storage to cover any possible shortage of wind and sun would be astronomically expensive. If we have to burn natural gas to meet 10% of our energy needs, that’s far better than what we do now, and buys us time to work on the last 10% (which would probably come from a storable carbon-neutral fuel).
Second, they give us a very helpful rule of thumb (bolded above) that we can apply at the regional as well as the global level: storage equal to an average day’s electricity usage will meet 90% of our demand, long-term. This rule is no substitute for a site-specific analysis with actual wind, solar, and demand data, but order-of-magnitude estimates are also useful.
What does this mean for the Capitan/PNM scenario? Earlier, I found that PNM customers use an average of 38 GWh of electricity per day. The rule of thumb says that matching that with 38 GWh of storage would get PNM down to 10% generation from fossil fuel (versus nearly 100% now). But the Capitan site only has 10 GWh of storage, so it’s too small by about a factor of 4, if what you care most about is getting to 90% carbon-free. Still, 10 GWh of storage will have a significant beneficial impact on PNM’s operations, and from the cost/benefit standpoint, we would expect that 10 GWh of storage to be very heavily used on a daily basis, so it should look good in terms of “bang for the buck.”
Now let’s apply the same rule of thumb to a much larger area: California. EIA has a dataset for the state as a whole. For 2019, the state’s total electrical demand was 179 terawatt-hours, for a daily average of 490 GWh1.
So, the rule of thumb says California needs 490 GWh of grid storage to get to a 90% carbon-free grid.
How much does the state have now? As usual, it’s not hard to find a power number: “California leads the U.S. in energy storage with 300 operational projects (7.2 GW).” No mention of the energy capacity, though. Many of those projects are for grid management (ancillary services) and of too short duration to help even out wind and solar.
Time for the usual sleuthing for energy figures; here is a lithium-ion project being built in San Diego County: “The Gateway project has a 15-year offtake agreement with Southern California Edison (SoCal Edison), and another long-term agreement with Pacific Gas and Electric (PG&E). Initially, the system will offer one hour of energy storage, increasing to nearly three hours in 2021 and to four hours later.” The power will “soon” be 250 MW, which at 4-hour duration would mean 1 GWh of storage from that project.
The same article discusses another project, Moss Landing: “Tesla and PG&E, though, have the option to upgrade Moss Landing’s capacity to as much as 1,200 MW, which Tesla has said could power every home in San Francisco for six hours.” That seems a bit more speculative, but assuming it happens, that’s another 7.2 GWh. The article goes on to say that “[a] project of that size would dwarf any existing battery storage project.” So it’s reasonable to think that the state’s battery total after these upgrades would be not tremendously larger than the 8.2 GWh the two projects will provide. California also has some legacy pumped storage projects from the 1970s and ’80s that are still in use. The rule-of-thumb goal of 490 GWh of storage for the state still seems distant. (But I’ll have more to say about that, a few posts down the road.)
RPSs (Renewable Portfolio Standards) As A Value Path
I’ll move on now from the question of how much storage we need, into the realm of how it might be paid for. As I mentioned, a number of states2 have passed renewable portfolio standards into law. These are legally binding now, but the goals are years in the future, so it remains to be seen how much societal pain (perhaps including drastically increased power bills, which are never popular) will be tolerated when it’s so much easier to just change the law once the going gets tough. In any case, if they’re made to stick, these laws will be a path to value for EPS, which Southwestern states will be able to use to meet their RPS requirements at the lowest cost to taxpayers and/or ratepayers. This means money will have to be allocated from state or other funds to build EPS plants.
That sums up the command-economy, “because we have to” approach: determine what we think needs to be built; pass a law mandating that it be built; and allocate funds. Simple (except politically).
What Is The Monetary Cost/Benefit Of EPS?
Now on to the market-economy angle. I deliberately confined my first look at costs to the realm of dollars; I set aside the non-dollar costs of EPS—the amount of carbon emission embodied in an EPS plant, the quantity of scarce materials it would use up, and so on—for another time. To be honest, the planetary costs of building, running, and eventually decommissioning an EPS facility are pretty trivial:
EPS borrows a small amount of steel (for penstocks, and for machinery inside the powerhouse, such as turbine runners, pump components, and so on), to be recycled into a different use eventually—but the amount is very small compared to the steel that goes into building a shopping mall. It borrows some copper, it borrows some water, it borrows some land that is generally not in use. It doesn’t need tellurium, like solar panels, or rare earth metals, like most wind turbines, or lithium or nickel, like lithium-ion batteries. The oil-derived plastic it uses may not be recyclable, but that borrowed carbon can, after many years of service, be buried deep in the ground to keep it out of our atmosphere. Anyway, the amount of plastic required is quite small compared to what we use for things like single-use beverage containers, and is also quite small relative to the job it does in harnessing water in order to reduce the burning of fossil fuels.
EPS does not require the mining, refining, and transportation of costly materials around the world, or the cleanup of environmental damage and wastes from acquiring them, aside from everyday materials that are already used in far greater amounts for more frivolous purposes. Decommissioning a EPS plant primarily means removing plastic bags from the surface, leaving no pollutants whatsoever to impair natural revegetation. The climate cost of EPS is almost embarrassingly small.
That leaves the task of quantifying the climate benefits of EPS, knowing that sooner or later, those must be mapped onto dollar benefits, or it won’t get built. Climate-wise, EPS exists solely to reduce carbon emissions.
EPS, or any electricity storage, only has significant benefits in the context of climate change. For those who don’t think climate change is a problem that we need to fix, the logical (or at least easiest) thing to do is to just keep burning more fossil fuels, and keep trying to find more of them. Coincidentally, that’s pretty much what we as a species are collectively doing. Ever since we figured out how to rebuild human civilization around fossil fuels, CO2 emissions have been on a one-way ride:
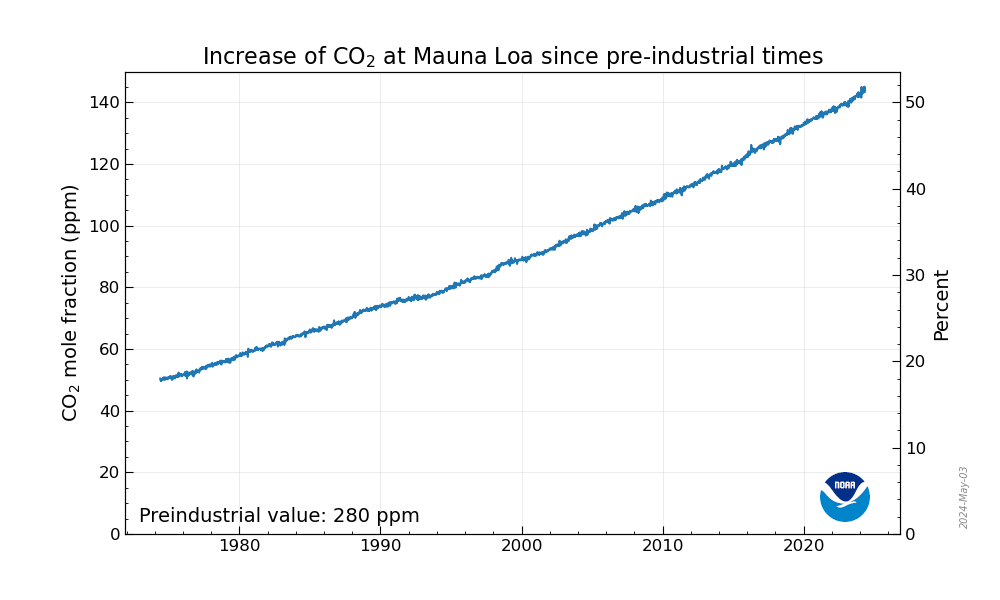
Carbon Taxes
I mentioned that the cost/benefit viewpoint naturally leads to proposing a tax on carbon emissions. There are some serious problems with a carbon tax (or a cap-and-trade scheme, which is a similarly market-oriented approach). One is administration: a carbon tax, like any other tax, creates a built-in incentive to cheat. The data collection required to detect and punish cheating is extremely complex and expensive, and even so, many cheaters (especially large ones) will get away with it.
Another problem with a carbon tax is a result of the economic distortion that our current, unprecedentedly skewed wealth distribution (in the U.S. in particular) creates. (Inequality.org states that “Three Men Own as Much as the Bottom Half of Americans“.) Regardless of how you feel about that as a moral issue, this much can hardly be questioned: Most of the decisions that have the biggest effects on CO2 reduction are made by corporations that are so wealthy that they can ignore losses for longer than political will (and tenure in office) can be sustained by those who want to use economic levers to make them change their behavior. So a carbon tax big enough to crush ordinary businesses and cause untold economic suffering could still be small enough for the billionaire class to brush aside as a routine cost of doing business. As a policy tool, it might not work as intended.
But my goal here is not to set social policy, but to quantify the cost/benefit relationship of EPS. For this purpose, an imaginary carbon tax, without these problems, will do. I picture it this way: every time you prevent one ton of carbon from being emitted into the atmosphere, a person from the government turns up and hands you a check for X dollars. The question, then, is what a reasonable value for X would be.
A 2018 Vox article by David Roberts says:
“As the low-hanging fruit is consumed, emission reductions start getting more expensive. To get to 80 percent (or more appropriately, 100 percent) reductions, carbon prices would likely need to exceed $100/ton by mid-century.”
I take this to mean that $100/ton is starting to get into the ballpark of representing the true cost of continued carbon emissions, even though it might never be politically feasible to impose a tax that high. (And “mid-century”? As in 2050? Surely we all know that our fates are sealed, one way or the other, long before then, don’t we?)
From https://energypost.eu/do-carbon-taxes-work/, emphasis mine:
“Perhaps the biggest challenge to make these plans work better is raising the per-ton tax to reflect new and higher forecasts for the future costs of climate change. These estimates will likely skyrocket within 25 years into hundreds of dollars per ton of carbon if the world is to keep the increase in global temperatures to less than 2°C compared to pre-industrial times, and an effective tax would need to be even higher for maximum warming of 1.5°C.
“That is far higher than the current average of about $20 per ton.”
This is an important observation and rings of truth. Setting a too-low carbon tax is one way we tacitly admit that we aren’t prepared to take the economic hit required to save ourselves in the future. (The carbon tax in the U.S. is, of course, zero.)
Let’s contextualize these carbon prices. We know how much CO2 is emitted when you burn natural gas, which has become the go-to fossil fuel for grid energy generation (coal’s on its way out, fortunately). This eia.gov article says natural gas emits 0.92 pounds per kWh.
(A good question to ask here is, pounds of what? carbon, or CO2? This is another one of those important questions that is not reliably answered. It matters: EIA also says that “assuming complete combustion, 1 pound of carbon combines with 2.667 pounds of oxygen to produce 3.667 pounds of carbon dioxide”. So preventing a pound of carbon from being added to the atmosphere is several times more beneficial than preventing a pound of CO2 from being added. You would think that writers would be more careful about clarifying which one they mean; some are, some aren’t. I’ll assume for now that 0.92 pounds of carbon, not CO2, is emitted when you generate 1 kWh of electricity by burning natural gas.)
Given this figure, if we assume society values not emitting a ton of carbon at $100, what is the societal value of preventing 1 kWh of electricity from being generated by burning natural gas? Switching to metric, 0.92 lb/kWh is 0.417 kg per kWh. Burning enough gas to emit a ton (1000kg) of carbon would generate 1000 kg / 0.417 kWh per kg = 2398 kWh or 2.4 MWh. So preventing a ton of carbon emission from natural gas is worth 100 dollars / 2398 kWh, or 4 cents per kilowatt-hour.
Put another way, a $100/ton carbon tax (much higher than those that exist now) would be society’s way of saying, “We want you to stop making those kilowatt-hours by burning natural gas, as long as you don’t spend more than 4 cents each doing it.”
That sounds pathetically small. Is it? Suppose you build 10 GWh of storage at the bargain-basement price of $75 per kWh. So your plant costs $750 million. And suppose that storage is kept so busy that it turns over its full capacity every single day, going from full charge to full discharge (which is unrealistic). That would prevent 10 GWh of gas from being burned daily, for a carbon tax “rebate” value of $400,000 each day.
It would take 1,250 days to pay off the cost of building the plant. That’s a bit under 3.5 years. Once paid off, the plant “earns” $400,000 per day. So 4 cents per kilowatt-hour doesn’t sound entirely hopeless after all.
If $100/ton is at the upper reaches of the politically feasible and the lower reaches of the climatically meaningful, let’s set that aside for now as an interesting number, one that we may be able to use later.
Modeling The Benefit Of Renewables And Storage
At this point, to get beyond rules of thumb, we need some simulation software. We can get hourly data for solar and wind energy at a particular latitude and longitude in the U.S. We can get hourly demand data for PNM. We just need to tie all this together. Hourly data matters because for storage, the devil is in the details: the time of day when the sun sets (ending solar energy for the day) relative to the time when customer demand spikes is of huge importance in determining what sort of storage is needed to prevent blackouts.
We’ll need an hourly model for energy inputs and outputs. We’ll also need a cost model for any system of solar panels, wind turbines, and energy storage that we might consider. Then we can start to look for solutions that seem both cost-effective and climate-effective. If it turns out that EPS just isn’t that good, then I’ll be honor-bound to propose that we don’t build any. Every scientist or engineer must be open to the frankly exciting possibility that their idea doesn’t work.
Previous: Encapsulated Pumped Storage, Part 12: Water
Next: Encapsulated Pumped Storage, Part 14: Modeling The Benefits
- This is 13 times bigger than PNM’s 38 GWh; California’s population of 40 million is 19 times bigger than New Mexico’s 2.1 million, so it seems Californians are somewhat more frugal with electricity. That’s supported by the figures at another EIA page, which isn’t directly comparable because it lists total energy use per capita, not just electricity; there, New Mexico is listed at 336 million BTU per person, and California at 202.
- An exhaustive list is at ncsl.org