Unsurprisingly, hydropower—the generation of electricity from water flowing in a river—preceded pumped hydroelectric storage, which is the use of water to store electricity generated from some other source than the river itself. The first commercial hydropower plant was built at Niagara Falls in 18791. Pumped storage began in 1907 near Schaffhausen, Switzerland2, so it’s a little younger, but still more than a century old.
The Golden Age of Hydropower
Skipping ahead to 1933, the first concrete was poured for the still-iconic Hoover Dam3. It’s all but impossible to imagine a project of this scale being built in the United States today. Power was first generated in 1936, and maximum power output gradually increased all the way until 1993, reaching over 2 gigawatts (2 billion watts).
Like all large hydroelectric generation (not storage) facilities, Hoover Dam was built by damming a river (the Colorado), in a location where the dam could trap a large amount of water with reasonable hydraulic head (elevation difference between the reservoir and the outlet). This takes a tremendous amount of concrete, because the bottom of the dam starts at the elevation of the original riverbed, so there is a great deal of hydrostatic pressure at the wet side of the base of the dam. That water at the bottom isn’t actually contributing—being at nearly the elevation of the outlet, if the lake ever drained to that level, it wouldn’t generate any power—yet it must be resisted.
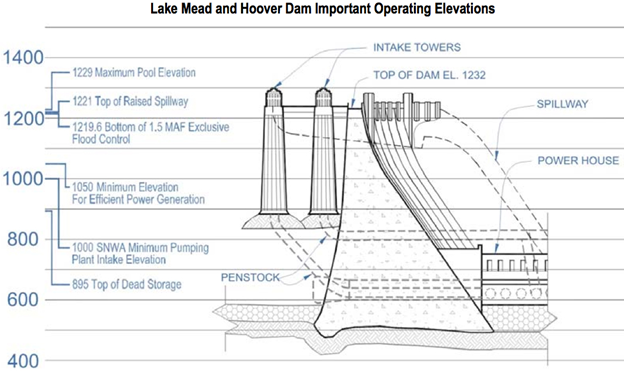
This diagram shows that the maximum pool (surface of Lake Mead when full) is at 1,229 feet (375m) above sea level, and the base of the dam is at about 500 feet (152m), a difference of 729 feet (222m). This would correspond to a pressure of about 300 pounds per square inch (20 atm) at the base of the dam4. The immense triangular wedge of concrete in the illustration is necessary to hold back all that force.
It’s instructive that Hoover Dam’s power output has been dwindling for years, due to lower river flows (persistent droughts to which climate change has contributed) and increased competition for water in the Colorado River basin. In 2014, the dam was reduced to operating as a peaking plant, only operating during times of highest demand for power5.
Adding Storage
A hydropower dam is inherently an energy storage device, but it doesn’t store electricity. It stores the gravitational potential energy inherent in the water that falls into its watershed as precipitation and flows into its reservoir from higher elevations upstream. (There are hydroelectric generators, called “run-of-river” facilities, that have no reservoir and don’t store energy; these are typically small, and lack the significant advantage of steady power output for as long as there’s water stored in the reservoir6.)
To turn a hydroelectric dam into a facility that can store electricity generated elsewhere, you need to add a second reservoir downstream from the first to capture the stream flow, instead of letting it escape. Then you install a pump at the lower reservoir, powered by electricity from some other facility. When that facility has surplus power, it can pump water back up from the lower to the upper reservoir, against the force of gravity. The energy this consumes can later be recovered when that water flows through the generators at the upper dam.
While this is an interesting hybrid of generation and storage, the age of damming large rivers is past, for reasons like wholesale destruction of riverine ecosystems and the carbon footprint of concrete dams.
Now that we’re talking about storing outside energy and not just generating it from the river’s own flow, let’s note some basic facts about pumped water storage. Assuming we are using water and not alternatives like molten lead, the density of our storage medium doesn’t change. So the amount of energy we store is (linearly) proportional to two quantities: how much water we move, and the elevation difference. Double the amount of water that can cycle between elevations, and you double the stored energy. Double the elevation difference, and you also double the stored energy.
Closed-loop Pumped Storage
A closed-loop system is not fed by a watershed; it uses the same water over and over (except for makeup water that is added, or allowed to flow in, to make up for water lost to evaporation or leakage into the soil). Some closed-loop systems use natural lakes for one or both reservoirs, sometimes impounding them to increase the volume of water stored. Though in particular cases this might be more acceptable than damming a river, this strategy is still subject to complaints about ecological damage, and new sites are scarce. So the new closed-loop systems that are being built now are more likely to build both reservoirs from scratch, in locations where this is politically feasible.
A pure example of this type of system, which is being built now, is the Gordon Butte Pumped Storage Hydro Project in Montana. Here’s a cross section:
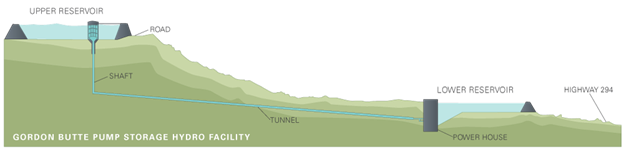
And here’s a bird’s-eye view:

For a system like this, we’d look for a site that has two reasonably flat areas, at different elevations, not too far apart horizontally. Then we’ll create a couple of artificial lakes, by some combination of digging, damming, and berming, as the terrain requires. We run a pipe with a turbine in it between the two ponds. Water is sent from the lower to the upper pond when we have surplus power to pump it, and goes down and spins the turbine when we want the power back later. (Though some turbine types, such as Francis turbines, can run backwards as pumps with good efficiency, there are sometimes reasons not to do this, but to keep the turbine and pump separate.) Because the amount of water is fixed, this system can store a finite amount of energy, like a rechargeable battery. It can only release energy when there’s water in the upper reservoir, and it can only store energy when there’s water in the lower reservoir.
Gordon Butte is located in a part of Montana that’s far from populated areas, in what most people would consider “empty land,” which generally means that it’s hard to extract any economic value from it. Aside from this advantage, the layout has other benefits. The flat terrain at top and bottom allow the water to be relatively shallow in each reservoir, which means we don’t need an enormously thick concrete dam—the shallowness of the water means hydrostatic pressures stay small, except in the penstock (the tube connecting the upper reservoir to the turbine, which is located inside the powerhouse near the lower reservoir). 300 psi of pressure was a big challenge at the base of Hoover Dam, due to the enormous area experiencing that pressure, but the penstock’s cylindrical shape and small size compared to a dam mean it can be strong enough to handle such pressures without costing too much. Meanwhile, the actual dams at Gordon Butte face far lower pressures, so there’s no need for monumental triangular wedges of concrete to hold the water back.
Gordon Butte is in an area that gets enough precipitation to fill the reservoirs naturally once they’re finished (we need enough water to half-fill each reservoir, since during operation, sometimes all of the water will be in the upper reservoir [the fully-charged state], and at other times it will all be in the lower one [fully discharged]). There is also enough inflow to make up for evaporation and seepage losses.
Gordon Butte, and other new systems like it, prove that I was oversimplifying when I described pumped hydro as a “built-out technology.” There’s every reason to believe this project will be a great success. The design is perfectly suited to its topography and climate. The price tag ($1 billion) is a little on the high side for what I calculate is 4.3 gigawatt-hours of storage capacity, but that price is only an estimate, and I’m not privy to the calculations that led to it.
Some of that cost may be due to another fairly cutting-edge aspect of the Gordon Butte design that I mentioned very briefly above. It’s a “ternary” system; that means that instead of having a single turbine that reverses itself to become a pump when surplus electricity is being stored, it has a dedicated turbine as well as a dedicated pump, both connected to a single electrical unit which is both a generator and a motor as required. Valves are used to change the routing of water through this equipment.
This mechanical/hydraulic arrangement has been around for some time, but it’s getting attention now because it offers the possibility of switching between storage mode and generating mode very rapidly (in a few minutes). This ability makes the pumped storage much more valuable in terms of overall grid operation. It also makes the storage more competitive with batteries (which, being purely electrochemical, tend to have very fast switching times).
There’s a lot more to say about how this ternary operation works, what it entails, and how it might interact with the particular features of Encapsulated Pumped Storage. That discussion will come later.
Previous: Encapsulated Pumped Storage, Part 1: Introduction
Next: Encapsulated Pumped Storage, Part 3: No Monolithic Reservoirs
- Wikipedia
- Wikipedia
- Wikipedia
- This source gives a figure of 45,000 pounds per square foot, which is 312.5 psi, so our numbers check out.
- Interestingly, in 2018, the Los Angeles Department of Water and Power (LADWP) proposed a $3 billion pumped hydro storage project that would use store wind and solar power, using Lake Mead as the upper reservoir. I will return to this later.
- Wikipedia