NOTE: This post does not reflect my latest thinking on water storage. Please see the new summary.
The Gordon Butte pumped storage design that we looked at in the previous post represents the current state of the art. Let’s continue into uncharted territory. Suppose we want pumped storage in a hot, arid climate, where evaporation losses from open reservoirs would be prohibitive. That means we need a waterproof cover—probably a floating plastic sheet, like a pool cover. (Tightly-packed floating balls are a possible alternative, but they tend to blow around the surface when it’s windy, and have other issues; I won’t consider them further.) Also, let’s assume that we want our reservoirs to be lined with an impervious membrane, so we can site them without worrying about seepage losses. We have now all but enclosed the water in a plastic bag, so let’s make that final step.
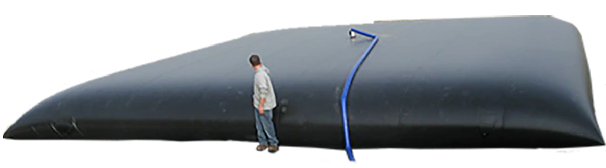
Large bags for liquid storage, called “bladder tanks”, “pillow tanks”, “onion tanks”, and so on (with variations in shape and design) are commercially available from many manufacturers. They’re made of flexible waterproof material reinforced with some sort of woven fiber. As seen here, these tanks are low in height relative to their length and width. This keeps internal pressures small and makes them self-supporting when placed on a flat surface.
As such a bag gets longer and wider at a constant height, the area of material needed for an array of bags approaches that needed for a pond liner and cover of the same volume. But there is no need for the reservoir to consist of only one bag. Instead, multiple bags can be interconnected with pipes to approximate the behavior of a continuous pond.
The humble word “bag” might not inspire confidence, with its connotations of flimsiness and disposability. What is a bag, in engineering terms?
A bag is a tensile structure. It doesn’t resist bending; it will readily assume any shape as long as its surface area stays the same at each point. And it isn’t damaged by bending: if you take a strip of sheet steel and fold it over onto itself, it will stay that way, permanently deformed; but a plastic bag will freely return to its original shape, unchanged. But it’s strong in tension, hard to stretch. When internally pressurized by a fluid (such as air or water), it will assume a minimal-energy shape that tends to equalize the tension within the bag material.
We can use these characteristics to our advantage in several ways. First, a bag like the one in the photo above can immobilize a large amount of water, and resist its pressure, relying only on the tensile strength of the bag material. So the amount of water that bag is restraining, relative to the amount of manufactured material comprising the bag, is enormous. And that is exactly the metric we need to maximize in any gravity-based energy storage system: control the largest possible amount of (free or cheap) heavy matter using the smallest amount of (expensive) manufactured material.
We also benefit from the fact that a flexible tank like the one above will conform to minor variations in the surface it’s sitting on, letting the terrain do much of the work of holding the water up. And a bag can collapse when drained—we don’t need to let air in as the water drains, and the bag doesn’t need to hold itself up when empty.
None of that would matter if we didn’t have suitable materials to make bags, but thanks to modern polymer chemistry and manufacturing, we do. This topic will be explored later, but for now, an anecdote about Neoprene (invented by DuPont in 1930), from the website of boatmaker Jack’s Plastic Welding: “Neoprene is renowned for its ability to last. When I was a guide in the Grand Canyon in 1979, outfitters were still using neoprene and canvas (cotton base fabric) tubes. This material predated the invention of nylon (before 1941). The reason they failed was because the canvas would rot inside of the neoprene, and they would lose their ability to hold air pressure. The coating was still OK.” 1
Grand Canyon rafts carry multi-ton payloads, and are dragged over rocks and exposed to intense sun, water, and temperature extremes. If a piece of material made before 1941 could endure that for nearly 40 years and still be “OK,” that suggests that the requirements for EPS can be met by one of the thousands of reinforced sheet materials that have been developed after Neoprene, or maybe even by Neoprene itself.
Besides preventing evaporation and leakage, what do we gain by storing the water in bags?
1. Our site preparation now only requires creating a flat and level area big enough for each individual bag. We don’t need to excavate a pond, or create one by raising berms or dams.
2. The water will stay clean. Our system will be immune to silt and debris, which are both problems with open reservoirs, particularly shallow ones, and especially if they have natural inflows, whether on a routine basis or during occasional floods that might overtop low dams. Existing hydropower plants devote a lot of effort to keeping things like fish and aquatic weeds out of the turbines and pumps. Even so, these are constantly exposed to some level of tiny particulates in the water, including hard materials like quartz, which steadily erode moving parts. Silt accumulation also slowly reduces the energy storage potential by reducing the effective reservoir depth, requiring expensive operations such as dredging to keep the plant in service.
3. In the event of a leak, we don’t have to try to do difficult underwater repairs, or drain the whole reservoir. Instead, we can drain the water from the affected bag into the rest of the system, close off the bag using a valve in its feed line, remove it, and replace it with a spare which can be immediately put in service. Then we can take the damaged bag to the shop for repair.
4. The bags can be mass-produced, making them less expensive than a custom-made pond liner and/or lid. This is also true of other water-handling components, like valves and fittings.
5. We can grow the capacity of our reservoirs over time by adding more bags, for as long as we have places to put them. We don’t have to take on the capital cost of building the largest reservoirs possible at the start of the project. This addresses one of the biggest shortcomings of pumped storage projects: the very long time between starting construction, and bringing the system online.
In spite of these advantages, I anticipate that this aspect of the EPS proposal—enclosing the water in bags—is likely to be the one that makes many people dismiss the whole scheme out of hand, based on an intuitive sense of implausibility, which could take many forms:
- You couldn’t contain a hydroelectric-scale quantity of water in bags;
- The bags will be too expensive;
- The bags will break down quickly because of sunlight, high temperatures, frost, hail, animal or bird attacks;
- The water pressure will burst the bags;
- The water will not flow in and out of the bags freely enough;
- and so on.
All of those points will be addressed at length in later articles. But theory aside, ultimately, only real-life testing will convince the skeptical, which is how it should be.
Ideal-world vs. Real-world Topography
Let’s look again at the Gordon Butte site:

The upper and lower reservoirs are clearly visible; the lower reservoir is in an area that is unmistakably flat. It’s not hard to convince oneself that a few passes with a bulldozer would be enough to prepare that area for an array of bags that would mimic the lower reservoir shown. And the upper reservoir also seems to sit in a location that needs very little work to achieve flatness.
Places like this are not especially rare in the American West, but they’re not really abundant either. And elevation enters into it: the elevation difference between the two reservoirs is roughly 1000 feet. I’ve found, with the help of Google Earth, that if you want more hydraulic head than that, the number of ideal sites diminishes quickly.
Because increasing the hydraulic head is the best way to make the energy storage cheaper, my general target is about 3000 feet (or 1 kilometer, more or less). There’s nothing magic about this number, because pumped storage is frustratingly linear, but more is certainly better. I would say that there are very few, if any, sites in the American West that have an upper storage area as flat and accommodating as the one at Gordon Butte, but at three times the hydraulic head.
Much of the intermountain West (not to mention other arid areas around the world) is “Basin and Range” topography, where material eroded from isolated mountain ranges (“Sky Islands”) has formed an alluvial plain. So what can be found, in relative abundance, are sites where the lower storage area is as flat as a billiard table, but the desired high elevations are not flat at all. If the site is far enough north to be glaciated, the mountains tend to be quite jagged, like the northern Rockies. A facility like Gordon Butte could not be built there, without blasting off an entire mountaintop in order to build the upper reservoir. (That is certainly possible, if money is no object, and the powers that be have committed to building that even if there are cheaper options—a scenario that seems unlikely.)
But south of the glaciated latitudes, there are many mountain ranges which have gentler, rolling tops. If we’re building our upper reservoir out of individual bags of water, is there some way we could exploit such terrain, which is somewhere between jagged and flat? The summary implied that there is; let’s explore that.
Suppose we have a mountaintop site that is only somewhat flat—it has slopes that would make digging or berming a reservoir difficult. If we use multiple bags instead of a reservoir, we only need a flat spot for each bag, but the bags can sit at different elevations. We aren’t looking for a huge amount of variation; we still want the upper and lower storage fields to be well-clustered around their respective average elevations. We just want to be able to use more of the terrain to store water. How can we make this work?
If the bags are simply interconnected by open tubes, it won’t work. The reason is that the whole system will behave just like a simple column of water. The water pressure at the top of the uppermost bag will be zero (in equilibrium with the atmosphere—this is called relative or “gauge” pressure—the absolute pressure is of course 1 atmosphere). Lower in that bag, pressure will be higher due to the weight of the water above. 10 feet (3m) down, at the bottom of the bag (numbers are for illustration only), there will be about 4 psi (0.27 atm) of extra pressure. Now move on to another, interconnected bag sitting at a lower elevation. The pressure keeps increasing as we go down in elevation, just as it did in the water behind Hoover Dam. After a modest 300 feet (100m) downhill, we’re at 120 psi ( 8 atm). A bag that can hold 120 psi will need to be thicker and sturdier—and thus more expensive—than the one at the top that only needed to hold 4 psi or so. So we’re losing our cost savings. (Much the same reasoning would apply to a pond 300 feet deep versus 10 feet deep.) The problem gets worse and worse as the variation within the upper storage area, the lower storage area, or both, increases.
Is there anything we can do to use the exact same cheap, low-pressure bags for the whole system, and still allow the bags to sit at different heights? Yes. We can put a pressure-limiting valve on each bag where it joins the piping system. Let’s say for example that our bags are rated for 10 psi (0.7 atm). We use a valve that will close any time the water in the bag is at 10 psi (gauge pressure). (This could be a passive, self-operating valve, or it could be electrically operated based on a separate pressure gauge inside the bag—that doesn’t matter for this discussion.)
Now imagine the network of bags sitting on the mountaintop, at somewhat different elevations. Initially the bags are empty and the valves are all open. As we pump water into the network from below, the lowest bag will fill first. Once it hits 10 psi, its valve will close. No matter what the pressure is in its feed pipe, the bag is isolated from that pressure and won’t feel it. It will sit safely behind its closed valve at the 10 psi it’s designed to handle.
As more water is pumped in, the second-lowest bag will fill. Its valve will also close at 10 psi and no more water will go in. The water will flow into higher and higher bags until it fills the uppermost one. When that bag’s valve closes, the upper storage will be at capacity and the pump will be shut off. Note that by this point, the pressure in the pipe adjacent to the lowest bag will be rather high, perhaps 40 psi in our thought experiment. Pipes, and valves, that can handle that sort of pressure are not expensive.
With this valve system, we’ll be able to store larger amounts of water for pumped storage, and more importantly, we’ll be able to exploit terrain with a very high hydraulic head, all without destroying entire mountaintops in the process.
Previous: Encapsulated Pumped Storage, Part 2: Historical Context
Next: Encapsulated Pumped Storage, Part 4: Some Storage Basics
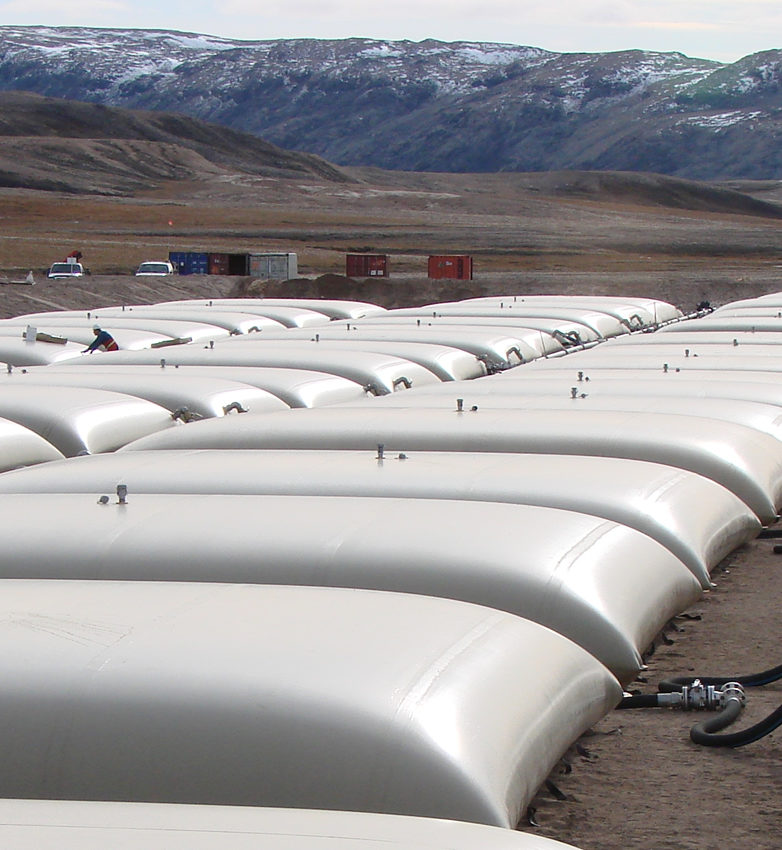